Black holes, with their immense gravity that engulfs even light, have captivated scientists for centuries. From John Michell’s initial proposition in 1783 to the elegant theory of general relativity by Albert Einstein, the concept of these cosmic behemoths has evolved from theoretical speculation to confirmed reality.
Today, thanks to advancements in computational power, scientists are delving deeper into the secrets of black holes, using exascale computing to unlock the mysteries of matter and energy in their vicinity.
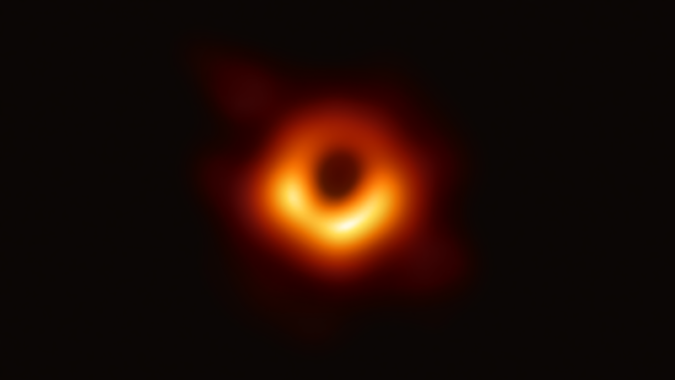
Black Holes: Powerhouses of the Universe
Black holes come in various sizes, with stellar-mass black holes being a few times the Sun’s mass and supermassive black holes tipping the scales at millions or even billions of solar masses. These cosmic giants are far from passive – as matter falls into their grasp, it transforms into superheated plasma, a state where atoms are stripped of their electrons. This infalling plasma doesn’t disappear silently; through a process called accretion, it releases tremendous amounts of energy, believed to power various phenomena within galaxies.
The Intricate Dance of Plasma and Magnetism
Dr. James Stone, an astrophysicist at the Institute for Advanced Study, is particularly interested in modeling the role of magnetism in black hole accretion. As the ionized particles in the plasma whirl around the black hole, they get caught in its gravitational pull. But magnetism plays a crucial role in this dance. The charged particles interact with magnetic fields, influencing their motion and ultimately affecting how the plasma spirals inwards.
General Relativistic Radiation MHD: Unraveling the Equations
To simulate these complex interactions, Dr. Stone and his colleagues employ a powerful tool – general relativistic radiation magnetohydrodynamics (MHD). This mouthful of a term essentially represents a mathematical model that incorporates the dynamics of plasma (MHD), the energy released as radiation, and the warping of spacetime due to the black hole’s immense gravity (general relativity). Solving these equations analytically, using pen and paper, is practically impossible. Here’s where exascale computing steps in.
Exascale Power: Unveiling the Black Box
The INCITE program, awarding access to high-performance computers (HPCs), has provided Dr. Stone and his team with the computational muscle needed to tackle these equations. Machines like Polaris at the Argonne Leadership Computing Facility and Frontier at the Oak Ridge Leadership Computing Facility, capable of performing a staggering million trillion calculations per second (exascale performance), are now instrumental in unraveling the mysteries of black hole accretion.
Athena++: The Code that Makes it Possible
The software powering these simulations is Athena++, a code developed by Dr. Stone’s lab over the years. This code tackles the complex MHD equations, but its true power lies in its adaptability. Thanks to the Kokkos C++ Performance Portability EcoSystem, Athena++ can seamlessly run on various machines, from laptops to these exascale giants. This portability is a game-changer, allowing scientists to leverage the ever-increasing computing power available.
Beyond Black Holes: A Code with Wide Applications
The beauty of Athena++ lies in its versatility. While currently focused on black hole accretion, the code can be applied to other astrophysical phenomena. For instance, Dr. Stone is using it to study cosmic rays, high-energy particles also produced by black holes, and their interaction with the turbulent magnetic fields in space.
A Legacy of Discovery: From Einstein to Exascale Computing
Dr. Stone acknowledges the transformative power of exascale computing, a tool that would likely have astonished even visionary scientists like Einstein.
These powerful machines allow us to probe the bizarre and fascinating realities surrounding black holes, pushing the boundaries of scientific understanding. As Dr. Stone concludes, “It’s amazing what nature does,” and exascale computing is giving us a front-row seat to witness its wonders.