For nearly a century, cosmologists have grappled with the mind-boggling concept of an expanding universe. First proposed by Georges Lemaître in 1927 and observationally confirmed by Edwin Hubble two years later, this idea has since become a cornerstone of our understanding of the cosmos.
Hubble’s groundbreaking observations revealed a critical relationship: the farther a celestial object resided from Earth, the faster it appeared to be receding, indicating a universe in constant expansion.
In 1998, a new twist emerged. Supernova observations by two independent teams painted a surprising picture – the universe’s expansion wasn’t slowing down as anticipated due to gravity; instead, it was accelerating. This revelation led to the now-dominant theory of dark energy, believed to comprise a staggering 68% of the universe’s energy, but its nature and properties remain shrouded in mystery.
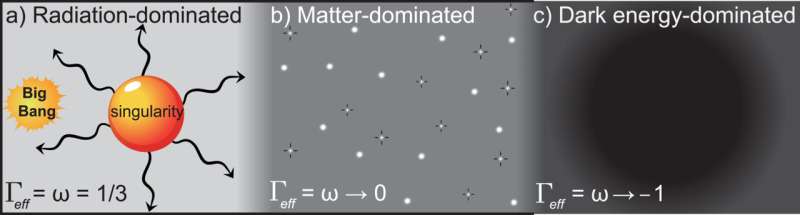
A Universe in Motion: Thermodynamics Enters the Cosmic Arena
Enter Professor Mariano de Souza and his team at São Paulo State University (UNESP), Brazil. Their research delves into the universe’s expansion using the principles of thermodynamics, the branch of physics concerned with heat and temperature. Measurements of the universe’s redshift (the stretching of light waves as celestial objects move away) suggest an expansion that’s both adiabatic (without heat transfer) and anisotropic (varying in different directions). Professor Souza explains, “These fundamental thermodynamic concepts imply that adiabatic expansion leads to cooling due to the barocaloric effect, a pressure-induced change in temperature.”
The Grüneisen Parameter: A Bridge Between the Infinitesimal and the Immeasurable
The concept of the Grüneisen parameter (Γ), introduced in 1908, plays a pivotal role in Souza’s work. This parameter quantifies the relationship between pressure, thermal expansion, and specific heat capacity, proving to be a valuable tool in geophysics. The research team builds upon the prior work of others who explored the behavior of the Grüneisen parameter near critical points in condensed matter physics, where materials undergo drastic changes in their properties.
A Novel Approach: Linking the Grüneisen Parameter to Dark Energy
Souza and his colleagues leverage the Grüneisen parameter to describe the complexities of the universe’s expansion. Their findings, published in Results in Physics, shed light on how the universe’s ongoing expansion is accompanied by continuous cooling. They propose a time-dependent nature for the Grüneisen parameter within the dark energy-dominated era (the current cosmological epoch).
One intriguing aspect of this research is the application of concepts from thermodynamics and solid-state physics, such as stress and strain, to the study of the universe’s anisotropic expansion. Professor Souza highlights, “Our work demonstrates a natural presence of the Grüneisen parameter within the energy-momentum stress tensor of Einstein’s field equations. This opens a new avenue for investigating the anisotropic effects associated with universal expansion, without necessarily negating the possibility of a Big Rip scenario.”
The Big Rip hypothesis proposes that under extreme circumstances, the accelerating expansion driven by dark energy could tear apart the very fabric of spacetime, shredding galaxies and ultimately ripping apart the universe itself.
Shifting Gears: A Thermodynamic Phase Transition in the Cosmos?
The research team further suggests that the shift from a decelerating expansion (during the radiation and matter-dominated eras) to an accelerating expansion (in the dark energy-dominated era) might resemble a thermodynamic phase transition. This analogy stems from the observed change in sign of the effective Grüneisen parameter (Γeff) as the expansion transitions from decelerating to accelerating, mirroring characteristic signatures of phase transitions observed in condensed matter physics, where a material abruptly changes from one state (like solid) to another (like liquid) under varying conditions.
Dark Energy and the Equation of State Parameter
Dark energy is often linked to the cosmological constant (Λ), a concept introduced by Einstein to achieve a static universe. While Einstein later abandoned this concept, the discovery of an accelerating universe led to its revival. The prevailing Λ-CDM model (Lambda-Cold Dark Matter) assigns a fixed value to the cosmological constant, implying a constant dark energy density throughout the universe’s expansion. However, some alternative models posit a time-varying dark energy density and, consequently, a non-constant cosmological constant.
Professor Souza explains, “Assuming a fixed value for Lambda translates to a fixed value for omega (ω), the equation of state parameter, a quantity used to describe the relationship between pressure and density in a substance. However, by recognizing omega as the effective Grüneisen parameter, we can infer a time dependence for omega as the universe expands within the dark energy-dominated era. This directly implies a time dependence for Lambda, or the universal gravitational constant.”
A Glimpse into the Future: Unveiling New Cosmic Secrets
This research holds immense
promise for revolutionizing our understanding of the universe’s expansion. By providing a novel thermodynamic perspective, it opens doors to further investigation using established principles from physics. Here are some potential areas of future exploration:
- Refining the Model: The team’s work lays the groundwork for a time-dependent Grüneisen parameter within the dark energy era. Future research could focus on establishing a more precise mathematical framework for this time dependence, potentially leading to a more nuanced understanding of dark energy’s behavior.
- Bridging the Gap Between the Microscopic and the Macroscopic: The application of concepts from condensed matter physics to cosmology offers a fascinating new approach. Further exploration in this direction could lead to deeper insights into how the universe’s expansion is influenced by fundamental physical laws governing matter at the smallest scales.
- Testing the Big Rip Hypothesis: While the research doesn’t rule out the Big Rip scenario, it provides a new lens through which to examine it. Future studies could leverage the thermodynamic framework to refine predictions about the conditions necessary for such a catastrophic event.
- Refining Dark Energy Models: The time-dependent nature of the Grüneisen parameter suggests a possible time dependence for dark energy density and the cosmological constant. This opens doors for exploring alternative dark energy models that incorporate this variability, potentially leading to a more comprehensive description of this enigmatic component of the universe.
Overall, Professor Souza and his team’s research presents a compelling case for employing thermodynamics to study the universe’s expansion. This innovative approach, coupled with further investigation, holds the potential to unlock a treasure trove of new knowledge about the cosmos, dark energy, and the ultimate fate of our universe.