Imagine a world where the microscopic and the macroscopic obey different sets of rules. That’s the current state of physics, where quantum mechanics reigns supreme for tiny particles and Einstein’s relativity governs the grand cosmos. But a new study proposes a solution to this perplexing divide, hinting at a way to unify these seemingly disparate theories.
The Quantum Conundrum: Superimposed States and the Paradoxical Puss
Quantum mechanics introduces the bizarre concept of superposition – the ability of an object to exist in multiple states simultaneously. Think of a coin spinning in the air; according to quantum mechanics, it’s both heads and tails until it lands. This principle works for tiny particles like electrons, but applying it to larger objects, like a cat in a sealed box with a vial of poison (Schrödinger’s famous paradox), creates a logical quagmire. The cat can’t be both dead and alive, can it?
The Challenge: Bridging the Quantum-Classical Gap
The crux of the problem lies in the seemingly incompatible nature of quantum mechanics and general relativity. While quantum mechanics thrives in the realm of elementary particles, the universe as a whole appears to follow the classical dictates of general relativity. Describing the entire cosmos using quantum principles seems nonsensical – there’s no external observer to “measure” its state, and superpositions seem improbable for such a vast system.
A Modified Maestro: The New Schrödinger Equation
Enter Matteo Carlesso and his team of theoretical physicists. They propose modifications to the very foundation of quantum mechanics – the Schrödinger equation. This equation dictates how quantum systems evolve over time, including those existing in superposition. Carlesso and his colleagues suggest adding specific terms to the equation, capturing how a system interacts with itself and nudging it towards a definite state.
The beauty of this modification lies in its targeted impact. Microscopic quantum systems, like atoms and molecules, remain largely unaffected. However, for larger systems, these additional terms act like a cosmic switch, triggering frequent collapses from superposition to a single, definite state. This naturally explains why the universe appears classical – frequent collapses ensure it exhibits well-defined properties, aligning with our observations.
Farewell, Superimposed Feline: Implications of the New Model
The modified model eliminates the need for an external observer. Instead, it posits that all systems undergo spontaneous collapses at regular intervals, acquiring definite values for specific attributes. For large systems, these collapses happen so often that they appear classical. Even subatomic particles interacting with these large systems get swept up in the collapse, rapidly acquiring definite properties.
This new framework offers a potential explanation for why spacetime, the fabric of our universe, doesn’t exist in a superposition of states. The model suggests that a quantum universe eventually collapses, effectively becoming classical. In essence, it proposes a mechanism for a quantum universe to transition into the classical one we observe.
The Road Ahead: Testing the Theory and Unifying Physics
While this theory offers a compelling explanation for the classical nature of our universe, it doesn’t predict new large-scale phenomena. Additionally, testing its predictions, particularly for microscopic systems where deviations from standard quantum mechanics are minimal, presents a significant challenge. Future research will focus on devising experiments to test these modifications and refine the model’s parameters.
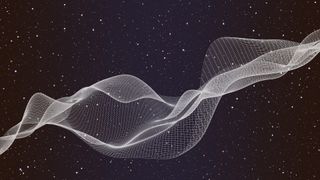
This quest to unify quantum mechanics and general relativity is a significant step towards a more complete understanding of the universe. By potentially explaining the emergence of classicality from a quantum origin, this modified model paves the way for a more unified physics, where the very small and the infinitely vast can be described under a single grand theory.