In the world of astrophysics, few events capture attention quite like gamma-ray bursts (GRBs). These powerful cosmic explosions release an extraordinary amount of energy and provide scientists with unique opportunities to test some of the most fundamental theories of physics. Recently, the brightest gamma-ray burst ever observed, GRB 221009A, has helped researchers place new constraints on quantum gravity, specifically testing Lorentz invariance. This discovery, made by researchers at the Large High Altitude Air Shower Observatory (LHAASO) in China, has far-reaching implications for our understanding of the universe and the very fabric of spacetime.
Gamma-ray bursts are known for their intense energy, and GRB 221009A stands out as the brightest and one of the closest such events ever recorded. Located approximately 2.4 billion light-years away, this burst offered scientists a rare opportunity to study high-energy radiation in extreme conditions. The brightness and proximity of this GRB made it an ideal candidate for testing quantum gravity theories, especially because of the unique chance it offered to measure the behavior of light traveling vast distances through space.
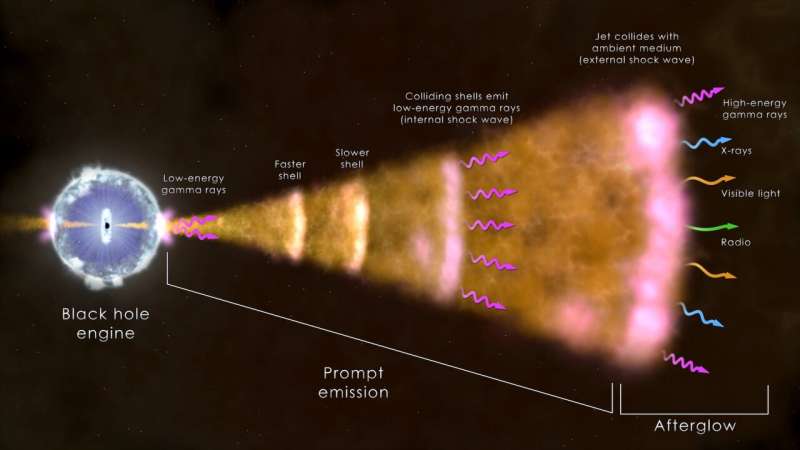
By analyzing the light from this burst, scientists hoped to detect tiny variations in the arrival times of different light frequencies, which would indicate a violation of Lorentz invariance. This principle, fundamental to Einstein’s theory of relativity, asserts that the laws of physics, including the speed of light, are the same for all observers, regardless of their motion. Testing this principle at such high energy levels allows us to explore the boundaries where general relativity may begin to break down.
The main focus of this study was to test Lorentz invariance, which is a cornerstone of general relativity. According to the theory, light should travel through a vacuum without any dispersion—meaning that all frequencies of light should arrive at the same time. However, some theories of quantum gravity suggest that at very high energies, space itself could behave like a medium, causing light to disperse similarly to how it does when passing through glass or water. This would violate Lorentz invariance and hint at new physics beyond Einstein’s equations.
Using data from LHAASO, scientists carefully measured the arrival times of different frequencies of light emitted by GRB 221009A. Remarkably, they found no evidence of dispersion, meaning that all frequencies of light arrived at the same time. This lack of observable difference allowed the team to place some of the tightest constraints yet on potential violations of Lorentz invariance. In particular, they were able to raise the energy threshold at which quantum gravity effects might emerge, narrowing the range where scientists should focus future tests.
The LHAASO team analyzed the energy spectrum of GRB 221009A over time, seeking any signs of frequency dispersion. They focused on the linear and quadratic terms that describe how light could potentially slow down or speed up depending on its energy. Previous studies of GRBs had already set limits on these effects, but the brightness and proximity of GRB 221009A allowed for more precise measurements.
For the linear term, which varies directly with photon energy, the team achieved results consistent with previous findings. However, for the quadratic term, they established a five- to sevenfold increase in the energy threshold where quantum gravity effects might become apparent. This represents a significant advancement in our understanding of how light and spacetime interact at extreme energies.
By placing tighter constraints on quantum gravity, scientists are refining the parameters for one of the biggest unsolved problems in physics: how to reconcile general relativity with quantum mechanics. These two pillars of modern physics work exceptionally well in their own domains—relativity explains large-scale phenomena like gravity, while quantum mechanics describes the behavior of particles at the smallest scales. However, unifying these theories into a single framework remains elusive.
The results from GRB 221009A help by narrowing the window where scientists should look for quantum gravity effects, guiding future experiments and observations. This discovery also highlights the importance of continued observation of gamma-ray bursts and other high-energy cosmic events. As technology improves and new observatories come online, scientists will have even more tools to probe the limits of our understanding of the universe.